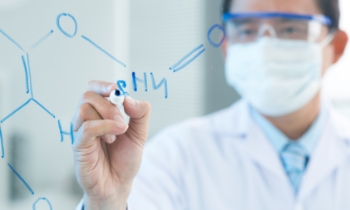
Precision in Practice: The Importance of Isolation and Purification in Impurities Profiling
In the realm of pharmaceuticals, precision is paramount. Every step of drug development, from synthesis to formulation, demands meticulous attention to detail. One crucial aspect often overshadowed by the spotlight on efficacy is the management of impurities. These unwelcome companions can compromise safety, efficacy, and even the integrity of the final product. To combat this threat, pharmaceutical scientists employ a combination of techniques, among which isolation and purification stand as pillars of precision.
Impurities come in various forms, from residual solvents and reagents to degradation products and contaminants introduced during manufacturing. Detecting and quantifying these impurities require sophisticated analytical methods, but before that, they must be isolated from the complex matrices in which they reside. This isolation process is akin to finding a needle in a haystack, albeit with potentially severe consequences if the needle is missed.
Isolation begins with careful selection of appropriate techniques based on the nature of the impurities and the matrix. Chromatography, the workhorse of analytical chemistry, finds extensive use in this regard. High-performance liquid chromatography (HPLC) and its variants, such as reversed-phase chromatography, provide excellent resolution and separation efficiency for a wide range of compounds. Solid-phase extraction (SPE) is another valuable tool, particularly useful for extracting impurities from complex mixtures like biological samples.
Regardless of the method employed, the goal remains consistent: to separate the impurities from interfering substances while preserving their chemical integrity. This is no small feat, as impurities often coexist with numerous matrix components, each vying for attention during the separation process. Achieving selectivity amidst such complexity requires a delicate balance of chromatographic parameters, such as mobile phase composition, column chemistry, and temperature.
Once isolated, the impurities undergo purification to remove any remaining contaminants and obtain a sample of sufficient purity for characterization. Purification techniques vary widely depending on the impurity’s physicochemical properties and the desired level of purity. Common methods include recrystallization, distillation, and liquid-liquid extraction, each offering unique advantages in terms of efficiency and scalability.
Recrystallization, for instance, exploits differences in solubility to selectively precipitate the impurity while leaving behind the bulk of the matrix components. This method is particularly effective for purifying solid impurities but may require optimization of solvent systems and cooling rates to achieve desired yields. Distillation, on the other hand, leverages differences in volatility to separate volatile impurities from non-volatile matrices. While highly efficient for volatile compounds, distillation may suffer from issues such as thermal degradation and entrainment of impurities in the distillate.
Liquid-liquid extraction occupies a middle ground, relying on differences in partition coefficients to selectively transfer the impurity between immiscible solvent phases. This method offers versatility in handling both solid and liquid impurities but demands careful consideration of solvent selection and extraction conditions to ensure optimal recovery and purity.
Regardless of the purification technique chosen, rigorous characterization is essential to confirm the identity and purity of the isolated impurities. Analytical techniques such as nuclear magnetic resonance (NMR) spectroscopy, mass spectrometry (MS), and infrared (IR) spectroscopy play pivotal roles in this regard, providing invaluable structural information and facilitating quantification at trace levels.
NMR spectroscopy, with its ability to elucidate molecular structures in solution, is particularly well-suited for identifying impurities with distinct chemical shifts or coupling patterns. MS complements this by offering high sensitivity and mass accuracy, allowing for precise determination of molecular weights and fragmentation patterns. IR spectroscopy, while less sensitive, provides valuable information on functional groups present in the impurity, aiding in structural elucidation and confirmation.
The combination of these analytical techniques enables pharmaceutical scientists to construct comprehensive impurity profiles, detailing the identity, concentration, and potential impact of impurities on product quality and safety. Armed with this knowledge, they can make informed decisions regarding process optimization, formulation adjustments, and regulatory compliance, ensuring that the final product meets stringent quality standards.
Moreover, impurity profiling serves as a crucial tool for troubleshooting process deviations and investigating unexpected changes in product performance. By tracing the origin of impurities and their pathways through the manufacturing process, scientists can identify potential sources of contamination and implement corrective actions to prevent recurrence. This proactive approach not only safeguards product quality but also enhances process robustness and efficiency over time.
In the broader context of pharmaceutical development, the importance of isolation and purification in impurity profiling cannot be overstated. These foundational steps lay the groundwork for subsequent analyses and decision-making, shaping the trajectory of drug development from conception to commercialization. By prioritizing precision in practice, pharmaceutical scientists uphold the highest standards of quality and safety, ensuring that patients receive medications that are both effective and free from harm.